By combining molecular self-assembly with electrochemical techniques, we create nanogaps that show fascinating nano-scale electronics and surface-chemical properties. Employing nanophotonic tools, we are able to unravel the fundamental nanoscale behaviour of light-matter interactions under applied bias or in electrochemical cells. Many areas are being explored, including self-assembled molecular optical switches, redox processes of 2D flatland materials, electro-luminescence of organic and quantum emitters, and probing ultra-low-energy optoelectronic nanodevices.
Current work:
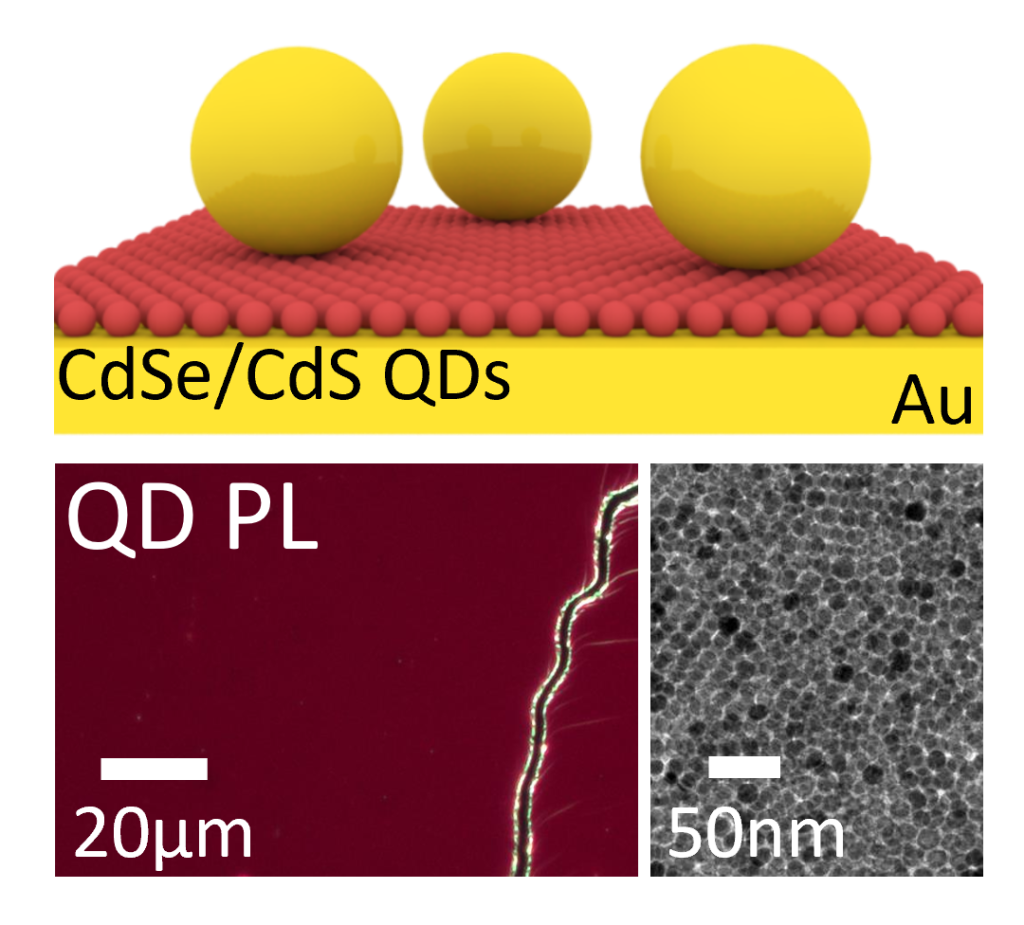
Coupling quantum dots in extreme nanocavities
Trapping light in volumes far below the optical wavelength dramatically increases the light-matter interaction. We have assembled semiconductor quantum dots into these ‘plasmonic’ nano-cavities which deeply changes how they emit and absorb light. We enter the strong coupling regime at room temperature where light and matter fuse together to create mixed states called ‘polaritons’, with unusual properties.
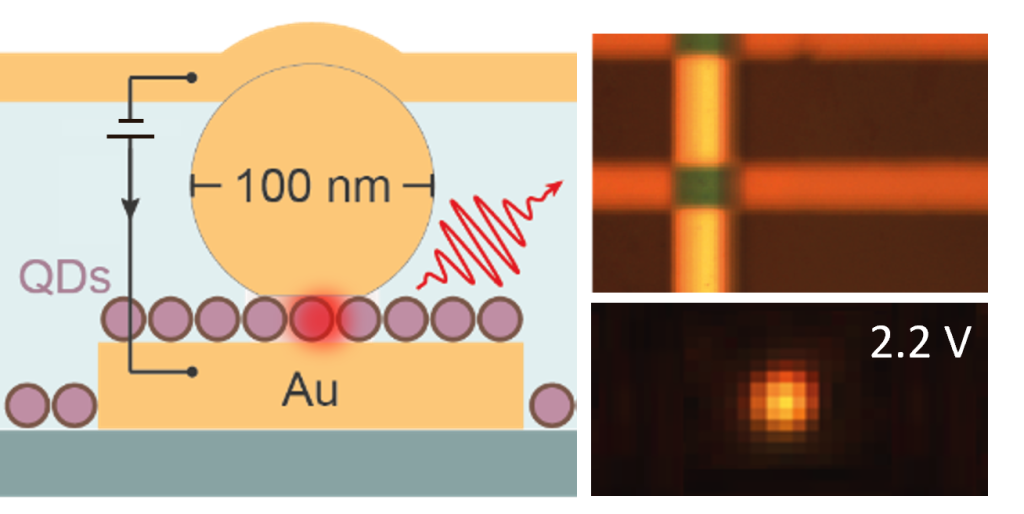
Electrically pumped QD LEDs in nanocavities
By integrating our devices using simple cheap self-assembly along with electrical contacts, we create LEDs from single quantum emitters. This opens prospects for quantum processing at room temperature. [1]
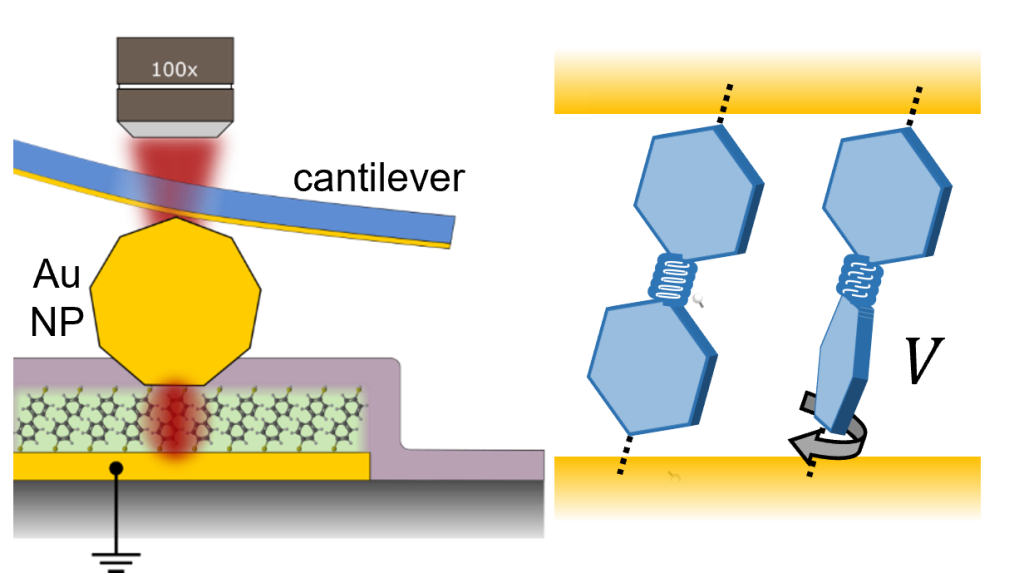
Watching molecules twist under electrical bias
By contacting the top of our nanoparticles which confine a single layer of molecules in the gap underneath, we create molecular electronics devices that can be probed by light. We find unexpectedly that at critical voltages, the molecules can twist, changing their conductivity. [2]
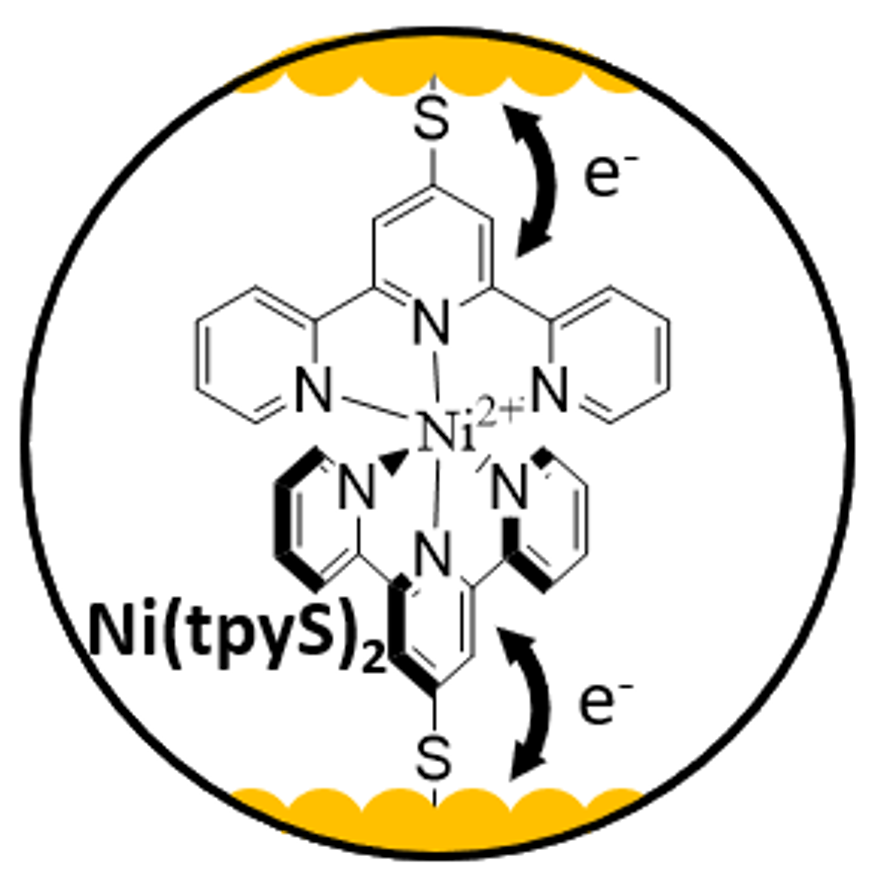
Watching CO2 catalysis in real time
By assembling catalysts for CO2 reduction in our nanocavities, we are able to use vibrational spectroscopy to watch the atomic processes in real time. This gives the capability to understand molecular electronics and catalysis in real devices, and improve them for applications. [3]
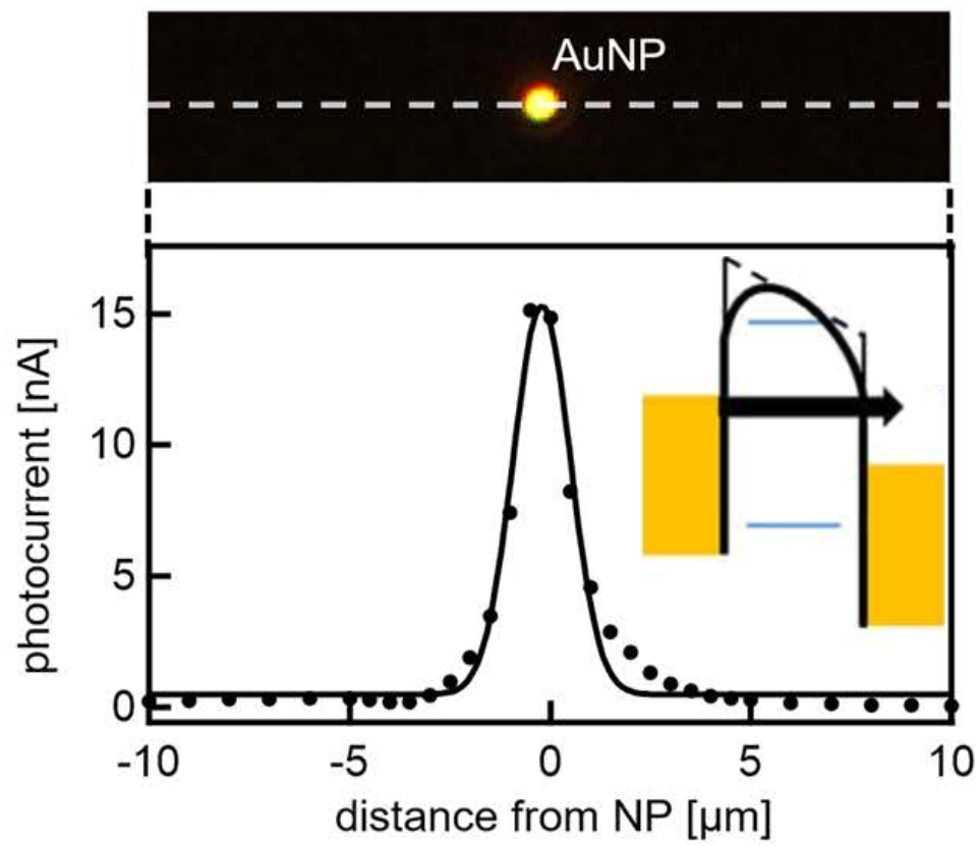
Light-induced quantum tunnelling of electrons
In our plasmonic nanogaps, the optical field is so intense that it can tilt the barrier enough to induce electron tunnelling. This photocurrent can be used as a nanoscale detector, and to explore the influence of quantum mechanics in optics. [4]
Key papers:
- Enhanced Photocurrent and Electrically Pumped Quantum Dot Emission from Single Plasmonic Nanoantennas, ACS Nano (2024); DOI: 10.1021/acsnano.3c10092
- Optical probes of molecules as nano-mechanical switches, Nature Comm 11:5905 (2020); DOI: 10.1038/s41467-020-19703-y
- Mechanistic study of immobilised molecular electrocatalyst by in-situ gap plasmon assisted spectro-electrochemistry, Nature Catalysis 4, 157 (2021); DOI: 10.1038/s41929-020-00566-x
- Quantum Tunneling Induced Optical Rectification and Plasmon-Enhanced Photocurrent in Nanocavity Molecular Junctions, ACS Nano (2021); DOI: 10.1021/acsnano.1c04100
Current people involved:
JJB, William Wood, Chenyang Guo, Zijia ‘Wendy’ Wu, Shijie ‘CJ’ Zhu